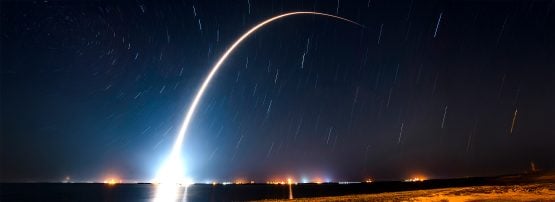
The way Starlink brings the Internet to you is through a clever system of microwave beams from its fleet of satellites. When a Starlink satellite is at least 25 degrees above the horizon, it can direct a focused Ku-band beam with a specific frequency and polarization at your ‘dishy’ (think of the beam having a particular colour of light). It’ll only stay on your dishy for a very short period. After that, it moves the beam away to service other users and then returns to your dish again in a round-robin fashion — all of this happens very fast, giving you the impression of a continuous connection.
So how does that impact the speed you experience? (Nag: The ‘speed’ is always the speed of light — what we really mean is the data rate, but let’s stick with the popular misnomer here). That depends on how long the beam can stay on your dishy and how quickly it can return to send more data. This process is similar to a teacher giving attention to students in a classroom. If there are more students, each gets less time, and it takes longer for the teacher to circle back. In this analogy, the satellite beam is the teacher’s attention, and the dishys are the students.
How to create extra capacity?
One way to create more capacity in a class is to reduce the number of students in it. For Starlink, this means having more satellites with more beams, but there’s a problem. They can’t point two different beams with the same frequency/polarization at the same spot, because then they’d interfere with each other — much like a student wouldn’t learn much having two teachers talk at them at the same time. There are only so many frequency channels in the spectrum — so what can be done?
Think of a beam as being a bit like a spotlight from a torch. If you move closer to a surface, the diameter of the beam also decreases. Similarly, if you put a big reflector on the spotlight, you can concentrate the beam on a smaller area. Both of these measures allow you to reuse the same colour closer by without the beams overlapping.
Bringing satellites down to a lower orbit moves them closer to the Earth’s surface and reduces the number of dishys within each beam’s coverage area. Moving satellites from 525km to 480km, for instance, decreases their footprint by about 16%, which could increase either the density of dishes they can collectively support or the amount of time they can dedicate to each dish, potentially improving ‘speed’ by around 20%. Using satellites with higher gain antennas also allows narrower beams, which can further improve this effect.
Another way to generate extra capacity is to transmit beams with higher power. Starlink uses OFDMA with QAM modulation — think of this in simplified terms as small differences in signal level and phase being used to convey information. A higher transmit power allows the satellite to accommodate more levels and phase values in the signal, while still allowing the dishy receiver to tell them apart. This translates into more bits per transmission. And Starlink has applied for permission to use higher beam power, too.
So, lower the orbit?
Lowering the orbit is a double-edged sword. Bringing the satellites down also reduces the number of satellites within view of each dishy. On the one hand, it reduces the risk of having a satellite with a spare beam that it can’t use, because the only potential clashes would now be between the satellites in view of the dishy’s cell, and there are now fewer of them. On the other hand, it increases the risk of not having a beam available at all when there is a dishy that needs service.
In the early days of Starlink, when there were much fewer satellites than now, not having a beam available was a far more serious problem. Even so, Starlink is considering relaxing the 25-degree elevation limit to 20 degrees, which would increase the number of satellites eligible to service a dishy by an average of nearly 43%. Conversely, each satellite becomes eligible to service a larger number of dishys.
The 25-degree limit was initially set to prevent Starlink satellites from transmitting interfering signals into the dishes of terrestrial microwave links, which are generally pointing within a few degrees of the horizon. Lowering this threshold could increase the chance of interference, especially in mountainous areas where terrestrial links may point uphill, which is why terrestrial providers like AT&T and Verizon have raised concerns in the past and are likely to do so again now.
What’s the catch?
The catch is that to enable all these improvements, SpaceX will need to replace its current satellites with larger ones, something they’re already planning since existing satellites have a lifespan of only five to six years. With SpaceX’s Starship launch system in development, launching replacement satellites should be even more economical, even though lower orbits mean increased drag, reducing satellite lifespans and raising fuel demands for collision avoidance.
I’ve personally seen download speeds up to 400Mb/s with the current technology on a roaming subscription. However, as more users joined, this capacity had to be shared in many places and speed declined despite the launch of significantly more satellites. Whether the proposed upgrades and orbital changes will receive the go ahead and be sufficient to keep up with growing demand remains to be seen.
Another aspect to remember is that the Internet is not just ‘speed’ as measured in speed tests — performance also depends on how close content is to the consumer, and how much of a bottleneck the path between content repositories and the consumer is. Like any Low-Earth Orbit system, Starlink has no means of putting content within a few dozen kilometres of consumers — something that many fibre users already enjoy. Moreover, where fibre users enjoy dedicated fibres on their last mile that have growth potential for many years to come, Starlink is reliant on shared physical radio spectrum for end user downlink. That shared spectrum can certainly be used more efficiently, as SpaceX propose here, but it doesn’t grow, and efficiency gains are comparatively small and hard-won.
Dr Ulrich Speidel is a senior lecturer in Computer Science at the University of Auckland with research interests in fundamental and applied problems in data communications, information theory, signal processing and information measurement.
The views expressed by the authors of this blog are their own and do not necessarily reflect the views of APNIC. Please note a Code of Conduct applies to this blog.